Cell Membrane Picture: Peering Inside a Living Cell Using Microscopes
Ask a cell biologist about their passion, and they might reveal a secret love for microscopes. After all, who wouldn't be captivated by the beauty of a cell membrane picture, a window into a world teeming with life?
This hidden wonder surrounds us, from the delicate patterns of a rose petal to the carrot in your salad. Every living thing, whether a blade of grass pushing through concrete or the skin on your hand, is a masterpiece made up of countless cells.
We, and the world around us are living cathedrals built of these tiny miracles. With the power of microscopes, we can step into these structures and marvel at the complex artistry of life itself.
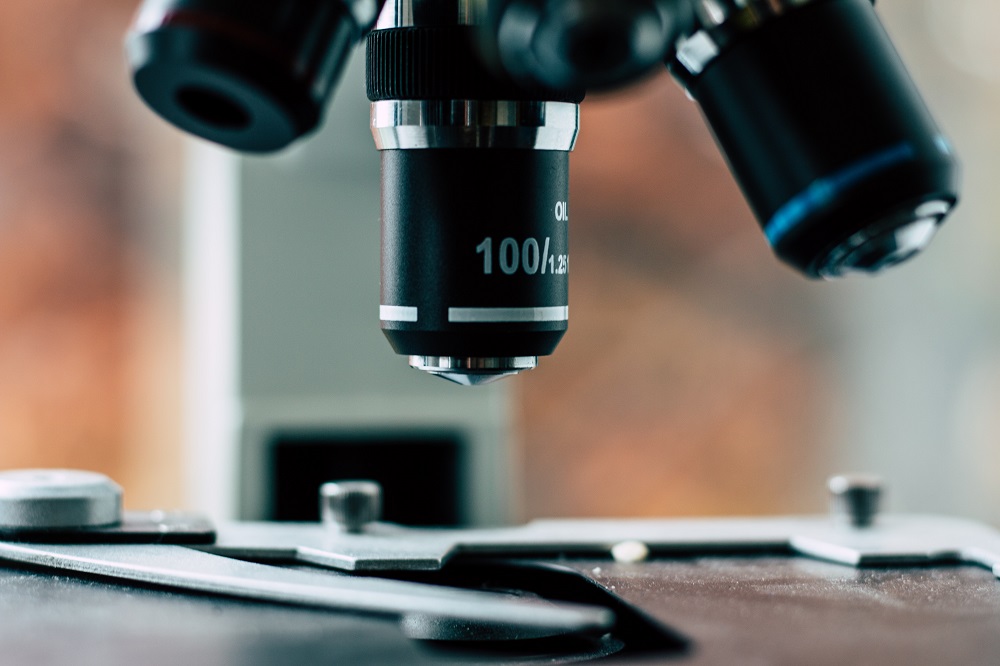
The Power of Magnification and Resolution
Cells come in various sizes, but they share one common trait: they’re all generally small. Take the human red blood cell, for instance. A single one is a mere eight micrometers (0.008 mm) wide. To put this in context, the head of a pin is roughly one millimeter in diameter, so you can line up 125 red blood cells in a row across it.
The human eye is incapable of seeing individual cells, with few exceptions. That's where microscopes come in. These instruments enhance our vision, revealing the details of cells that would otherwise remain hidden.
While a magnifying glass might seem like a simple tool, it's actually a basic microscope with a single lens. More sophisticated compound microscopes use multiple lenses, cleverly arranged to bend light and produce highly magnified images.
So, what sets a basic microscope apart from the powerhouses in research labs? Two crucial factors: magnification and resolution.
Magnification dictates how much larger an object appears. A typical light microscope found in schools can enlarge objects up to 400 times their actual size. On the other hand, resolution determines the level of detail we can see. It's the ability to distinguish two close objects as separate entities. High resolution allows for sharp, detailed images that show the intricate structures within a cell membrane picture.
Think of it this way: High magnification without good resolution is like enlarging a blurry photo—it's bigger but still unclear. Both magnification and resolution are essential for capturing the hidden wonders of the cellular world.
Electron Microscopy
Having established that different microscopes vary in magnification and resolution, let’s focus on the type that can aid you in peering inside a cell.
Electron microscopes are some of the most powerful kinds of microscopes. They are capable of distinguishing even individual atoms. However, as powerful as they are, you can’t use them to image living cells because the electrons destroy the samples. Recent advancements in electron microscopy have allowed scientists to get a better look.
Advancements in Electron Microscopy
Recent innovations, like transmission electron microscopy (TEM) combined with sealed liquid chambers, have brought scientists closer to visualizing living cells at the nanoscale. Imagine capturing a high-resolution cell membrane picture in real time, witnessing the dynamic molecules within.
The challenge remains in distinguishing between a cell's natural state and potential alterations caused by the electron beam. Are the observed changes in a cell membrane representative of reality, or are they artifacts of the imaging process? The question remains open, but progress is underway.
Dynamic Imaging
Traditional TEM requires samples to be lifeless, existing in a vacuum within the microscope. On the other hand, Liquid cell TEM allows scientists to observe biological samples in their near-natural, liquid environment. This opens doors to studying the behavior of molecules within a cell membrane, offering a glimpse into the activity within.
But, limitations still exist. Each sample requires a separate chamber, making it difficult to compare and validate observations. The risk of electron beam damage persists, raising questions about the accuracy of the resulting cell membrane image.
Better Beams
Progress isn't limited to sample preparation. Advances in electron beams themselves are pushing the boundaries of microscopy. By refining the energy of electron beams, scientists can look deeper into the atomic fabric of materials, mapping not only structures but also their properties.
For instance, by observing atomic vibrations (phonons) in a cellular membrane, researchers can uncover clues about how cells interact with their environment and respond to stimuli. These insights have far-reaching implications, from understanding disease processes to developing new materials.
The Future of Electron Microscopy
Electron microscopy has come a long way since its inception, but the ability to image liquids has always been a challenge. Enter liquid-phase electron microscopy, a rapidly growing field that's transforming how we visualize molecules in their natural, fluid state.
Unlike traditional electron microscopy, which relies on fixed or frozen samples, liquid-phase electron microscopy allows us to observe the dynamic movement of molecules in real-time.
This opens up a wealth of possibilities, from studying chemical reactions as they unfold to observing the intricate interactions within a living cell membrane.
Key Takeaways
The future of microscopy is unfolding before our eyes. From unveiling the secrets of cell membranes to driving breakthroughs in materials science and medicine, this technology is set to reshape our understanding of the microscopic world.
Ready to explore the possibilities of electron microscopy for your research? We offer cutting-edge solutions tailored to your needs. Contact us to learn how we can help you maximize your research impact and equipment investment today!